
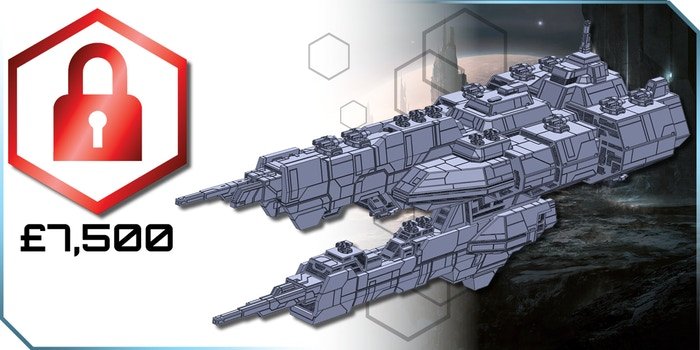
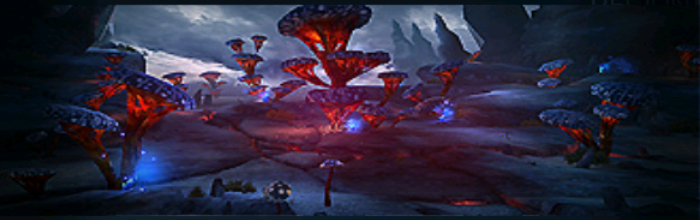
Binary stellar systems give rise to nova explosions, X-ray bursts, type Ia supernovae, neutron star, and neutron star–black hole mergers. This involved improvements in stellar models, the composition of stellar wind ejecta, the mechanism of core-collapse supernovae as final fate of massive stars, and the transition (as a function of initial stellar mass) from core-collapse supernovae to hypernovae and long duration gamma-ray bursts (accompanied by the formation of a black hole) in case of single star progenitors. Since then a steady stream of nuclear experiments and nuclear structure theory, astrophysical models of the early universe as well as stars and stellar explosions in single and binary stellar systems has led to a deeper understanding. The papers by Burbidge (Rev Mod Phys 29:547–650, 1957) and Cameron (Publ Astron Soc Pac 69:201, 1957), as well as precursors by Bethe, von Weizsäcker, Hoyle, Gamow, and Suess and Urey provided a very basic understanding of the nucleosynthesis processes responsible for their production, combined with nuclear physics input and required environment conditions such as temperature, density and the overall neutron/proton ratio in seed material. Astrophysical environments from the Big Bang to stars and stellar explosions are the cauldrons where all these elements are made. in magnets or lasers, lead or lithium in batteries, and also of naturally occurring uranium and plutonium? The answer lies in the skies. What is the origin of the oxygen we breathe, the hydrogen and oxygen (in form of water H 2O) in rivers and oceans, the carbon in all organic compounds, the silicon in electronic hardware, the calcium in our bones, the iron in steel, silver and gold in jewels, the rare earths utilized, e.g.
